Лаборатория геологии техногенных процессов
Maximovich N. G., Kazakevich S. V., Hmurchik V. T. Development of methods protection of the Kama reservoir from oil pollution // Quality and management of water resources: 3rd Symposium : book of proceedings. St. Petersburg, 2005. P. 362-369.
DEVELOPMENT of METHODS of PROTECTION of the KAMA RESERVOIR from OIL POLLUTION
Maximovich N. G, Kazakevich S. V., Hmurchik V. T.
During fifty years period of exploitation of Polazna (Western Urals) oil deposit lens of oil was formed on the surface of subsoil waters and became a source of pollution of Kama Reservoir. The analysis of geological and hydrogeological information on oil deposit has shown that discharge of oil on a coastal line is connected with its long-term exploitation.
The available data show that the most probable and basic source of technogenic oil lens forming are spills and discharge of oil on the ground surface at initial stages of exploitation of deposit, and a major factor promoting this intensive karstification of the territory.
For pollution control of Kama Reservoir two methods taking into account complex geological and ecological conditions of the deposit were offered: pumping of oil from the surface of lens with the special technology; biochemical destruction of oil on the basis of activization of microorganisms existing in lens of oil and participating in its natural biodegradation.
The hydrosphere at oil deposits in places with highly karstified surface is vulnerable to pollution. Oil production and refinery impact on the environment is significant. Contamination with oil products, surface active agents and various chemical reagents alter the composition of ground and surface water as well as soils and rocks. Misoperation of boreholes can disturb the hydrodynamics and hydrochemical regime of ground water and can favor its overflow from one aquifer to another. The areas of oil deposits are the most vulnerable to transformation.
The Perm region (the Western Urals) is one of the areas of oil-deposit development. Oil deposits occupy vast areas in the Perm region, both southern and northern parts. A considerable number of them is located within the drainage area of the Kama River. In this area, the groundwater is poorly protected from pollution due to the intense karst development (Fig. 1) [1]. Contaminated groundwater is discharged to the water reservoir and causes a significan pollution of water reservoir and adjacent areas. The Polaznenskoe oil deposit is an example of such an area, where oil lenses were formed at the groundwater surface during its half a century development. These lenses contaminate water of the Kama water reservoir.
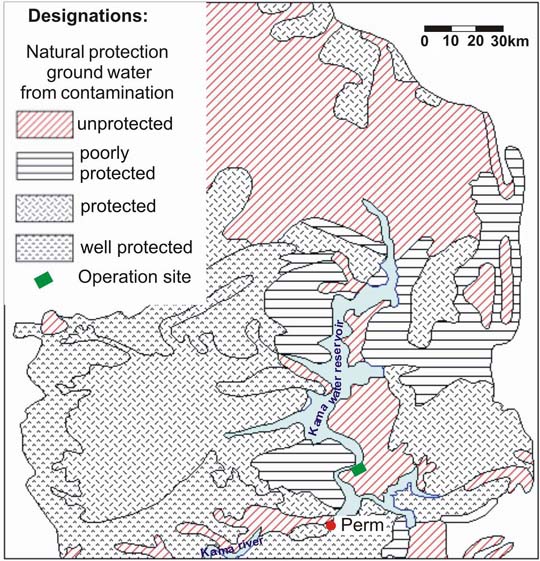
The analysis of geological and hydro-geological information proved that oil discharge to the coast is related to the long-term operation of oil deposit. The intense karstification of the area appears to be one of the principal natural factors that provided the development of oil pollution source at the surface of fracture-karst water [1, 2, 3].Perm region occupies an area of about 160 thousand sq. km and is located within three major geological structures, i.e., the eastern margin of the East European platform, the Pre-Urals foredeep, and the fold belt. The karstified rocks, i.e., limestone, dolomite, gypsum, anhydrite, and Paleozoic rock salt, are either exposed or lie close to the surface in the area of about 30 thousand sq. km. The deposit area is situated in the central part of the Perm region within the Polazninskii site of Polazninskii karst region (predominantly of gypsum and carbonate-gypsum karst) [4]. This area encloses the left coastal side of the Kama water reservoir in the vicinity of the Polazna settlement. Thickness of gypsum-anhydrite stratum of irenskii horizon is higher than 100 m, with either absent or thin mantle rock. Karst is formed of typical sulfate compounds. The specific feature of this site is its intense karstification. The average density of karst forms is 60 units per sq. km, at some microsites it reaches the value of 5001000 (Fig. 2). 1691 karst forms are mapped within the area of 28,1 sq. km, 97% of them are sinkhole (Fig. 3) [5, 6].
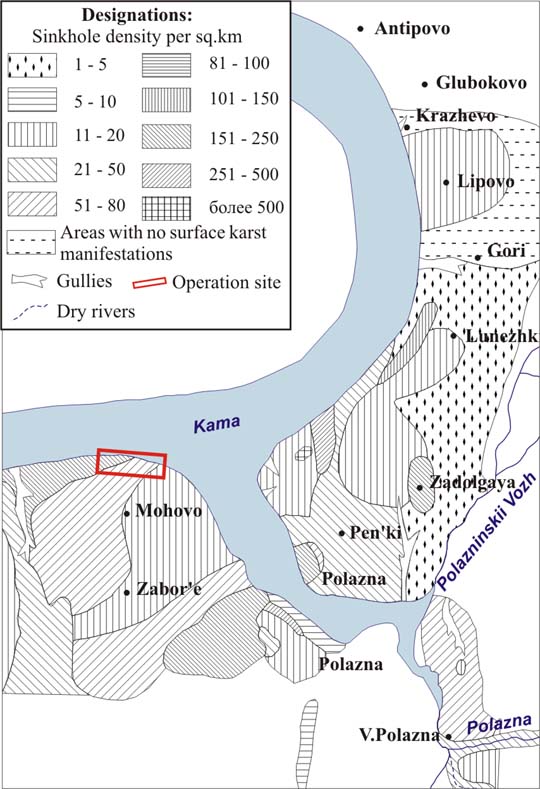
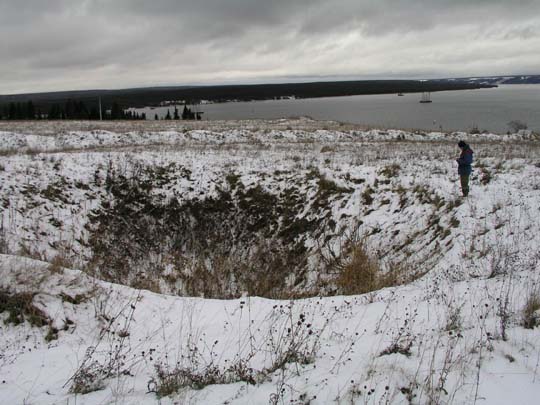
The site with the oil lens is in the Mokhovskoe karst field. According to geomorphology, it represents an uplifted slightly wavy plain abruptly breaking to the water reservoir and slowly sloping to the gulf. The depth of the local base level increases up to 6080 m towards the steep slope of water reservoir, in the direction of the southeast of Zabore village in particular (80100 m).
Karst development in the region was promoted by formation of the Kama water reservoir in 1954, which induced the water level rise by 2022 m. Filling the reservoir with water up to the project level in spring and falling of the water level by 68 in winter changed the hydrodynamic zoning of karst water and favored the recurrent ingress of weakly mineralized water to the rock massif [2, 6, 7].
Thus, the studied site is specified by a number of peculiar features caused by karst development, which provides contamination with oil products of the uppermost aquifer. Above all, the surface runoff is virtually absent. Atmospheric precipitation as well as oil spills are freely absorbed by rock fractures, sinkhole, depressions and other karst forms. This is a specific feature of the given oil deposit. At other oil deposits, where the environmental conditions are different, the oil spills contaminate, above all, the surface water, soil and rock in the aeration zone, and the ground water.
Karst produces considerable filtration heterogeneity of the rock massif. The bulk of karst cavities occur within the zone of seasonal fluctuations of fractural-karst water. After oil penetrates into this zone, we can conventionally presume the formation of some kind of human-induced oil deposit in karst collectors. As was mentioned above, construction of the Kama reservoir has intensified karst processes. This provided even more favorable conditions for pollutant percolation into karst water.
The regime observation in boreholes was performed for investigation of oil lens. This monitoring testified a similar water level in the Kama water reservoir and boreholes drilled 50400 m far from the shore, as well as their close hydraulic relationship due to high level of rock karstification. The study of groundwater chemical composition in boreholes showed sulfate-hydrocarbonate-calcium composition of groundwater and its mineralization that ranged from 2,2 to 3,0 g/1.
The region of contaminated groundwater discharges to the Kama water reservoir is grossly heterogeneous. There are three zones of intensive groundwater discharge, for example, springs in winter (Fig. 4) and subaquatic way in other seasons. Mineralization of the near-bottom water in anomalous zones amounts to 0,53,5 g/1. Outside of the intensive discharge zone, mineralization range is 0,10,4 g/1.
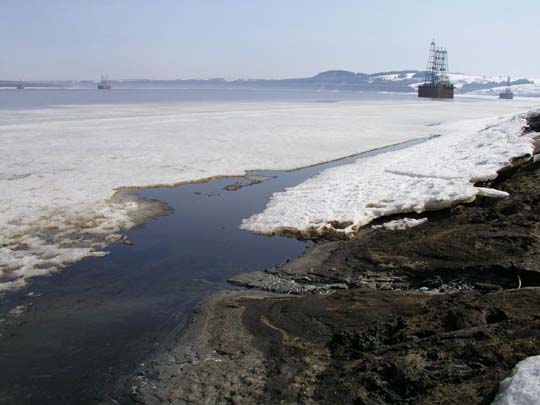
Two methods of pollution control in the Kama water reservoir were used. These methods considered the following geological and environmental conditions at the deposit: pumping oil from the lens surface using special technique and biochemical destruction of oil by microbial activation in oil lenses using natural biodegradation.
The groundwater provides a habitat for prokaryotic and eukaryotic microorganisms alike, but bacteria are the most abundant. Nowadays, groundwater microbes are systematically studied. A great number of subsurface environmental factors can influence microbial activity and, therefore, transformation of pollutants. These factors are temperature, pH, redox potential, availability of electron acceptors, salinity and hydrostatic pressure, porosity of the aquifers rocks, chemical recalcitrance and solubility, chemical and physical adsorption and desorption on rock particles. Because the habitat may differ for different geographic regions and subsurface depthes, a large variety of different microorganisms was detected and characterized. About 106107 bacteria per gram were recovered from the vadose zone and some shallow-watertable aquifers. The number of free-swimming bacteria was 10100 times lower [8, 9, 10, 11, 12]. Microbial constitution of sediment samples is more diverse than constitution of water samples, because chemical composition of groundwater samples is more homogeneous than that of sediment ones [11, 12].
Although groundwater is more difficult to be contaminated than surface water, reclamation of contaminated aquifers is also aught to be much more difficult. Improved analytical capability revealed widespread presence of toxic waste chemicals in groundwater. Nowadays, many world aquifers that contain vital groundwater supplies are polluted with toxic chemicals [10]. A significant variety of microorganisms found in groundwater are able to transform not only natural organic compounds under aerobic and anaerobic conditions, but also a large number of xenobiotic contaminants. In general, contaminated groundwater contains acclimated microbial populations able to transform pollutants under the existing redox conditions and, therefore, pathways of pollutant transformation can differ for various redox conditions. The presence of sufficient electron acceptors will be a principal factor in predicting the degradation of organic contaminants in aquifers. Usually pollution has a stimulatory effect on the growth of groundwater microorganisms at concentrations below toxic levels. When pollutant concentrations reach high levels, microbial metabolism can be inhibited. In uncontaminated groundwater oligotrophic conditions prevail and, therefore, sufficient oxygen concentration is be present. However, anaerobic microorganisms can be isolated in such environments, indicating the existence of special microhabitats that allow anaerobic microorganisms to survive in these areas. Recently, several reports have indicated that groundwater microorganisms are able to degrade organic pollutants under anaerobic conditions [13, 14, 15, 16, 17, 18]. When excess of organic pollutants leach from the surface into groundwater, inorganic nutrients, especially nitrogen and phosphorus, are to be limited, and many compounds are to be degraded only partially. Other elements, like magnesium, potassium, calcium and trace elements necessary for microbial growth, are usually available in concentrations high enough to support microbial growth [9, 10]. Field studies show that contaminant biodegradation processes in aquifers can be controlled largely by site-specific geochemical and hydrogeologic factors [19]. Because oxygen transport limitations can retard the aerobic biodegradation of pollutants in groundwater, anaerobic processes can often determine the fate of pollutants [9, 17]. When the biodegradation rates of various organic compounds have been measured employing various alternate electron acceptors (nitrate, sulfate, and carbonate), the rates of transformation were relatively rapid compared to aerobic rates [15, 14, 17]. McNabb and Dunlap have postulated that essentially all naturally occurring organic compounds are utilized by microorganisms under suitable growth conditions [20]. Microorganisms in groundwater are active in situ, and therefore play a significant role in influencing groundwater chemistry and quality [21]. And it was recognized that
groundwater biological activity can provide the cleaning up of polluted aquifers [11].
The most common pollutants of groundwater are hydrocarbons and heterocyclic compounds of oil and oil-products. There are two main biotechnological methods of oil polluted natural habitats remediation: 1) stimulation of natural microbial hydrocarbon-oxidizing populations by nutrient supplies (especially nitrogen and phosphorus), and 2) introduction of active hydrocarbon-oxidizing bacteria (and nutrient supplies) into polluted environments.
In this study we tried to combine these approaches in order to achieve the cleaning up of oil-polluted karstic aquifer. This work consists of the following several stages: isolation of active hydrocarbon-oxidizing bacteria from karstic groundwater and study of their oil degrading capability; development of bacterial preparation based on isolated aboriginal hydrocarbon-oxidizing bacteria to remediate oil polluted groundwater; stimulation of hydrocarbon-oxidizing microflora of aquifer by inorganic nutrients supplies; and introduction of the developed bacterial preparation into aquifer to achieve complete oil removal. Noteworthy that introduction of actively metabolizing hydrocarbon-oxidizing bacteria into polluted environments is essentially actual in regions of cold and temperate climate, where warm season is short.
In this investigation, capability of groundwater microbiota to degrade oil in the laboratory correlates with capability of natural groundwater microbiota to degrade oil. A sample of groundwater was aseptically collected in a sterile glass bottle, capped with an aluminium cap and stored on ice during transportation to the laboratory. All manipulations were carried out during 24 hours. Experiments were carried out in 250-ml flasks, containing 50 ml sterile medium: (NH4)2SO4 2 g; KH2PO4 0,4 g; Na2HPO4 0,6 g; microelements solution 2 ml, crude oil 2% vol.; H2Odistilled 500 ml; H2Owater-supply 500 ml. Two test flasks were inoculated with a groundwater sample (10% vol.), two control flasks were not inoculated. All flasks were incubated for 2 weeks at room temperature in a rotary shaker (90 r.p.m.) and for 2 weeks at room temperature without any shaking. After that, GLC- and IRS-analysis of oil remained in test and control flasks was made.
Infra-red spectroscopic analysis revealed the decline of peak intensity of aromatic rings at 1600 and 875750 cm-1, the decline of CH2- and CH3- groups peak intensity at 2900, 2800, 1470, 1380, 720 cm-1, and the decline of various C-O bonds peak intensity at 17401700, 1260, 1090 cm-1 both in test and control flasks. But these declines were more distinct in test flasks and weak in control ones. The decrease of К and C3 spectral coefficients, and the increase of C1, C2 and K1 spectral coefficients were observed in test flasks. This can be accounted for the decrease of the amount of n-paraffinic compounds.
Gas-liquid chromatographic analysis revealed a 4-fold decrease in total n-alkanes (Σn-C1234) concentration in test flasks related to control ones. The significant change in n-alkanes and naphtenes ratio was observed in test flasks: value of n-C18/napht, n-C24/napht, and n-C30/napht coefficients decreased in two times. The ratio of n-alkanes and isoprenes in test flasks increased in five times (coefficients Σi-C1420/Σn-C1234 and Ki=Σi-C1920/Σn-C1718). The changes in n-alkanes ratios in test flasks (Σn-C1518/Σn-C1922, Σn-C1723/Σn-C2430, Σn-C<21/Σn-C>20) showed that groundwater bacteria metabolized oil hydrocarbons of small and medium molecular weight without any preference of «even» or «odd» n-alkanes.
As a whole, due to groundwater microbial metabolism the content of n-alkylic compounds in oil decreased in four times. At the same time, the decrease of these substances in control flasks due to physical and chemical factors doesnt exceed the value of 20%. Microbiological investigation of karstic groundwater revealed the presence of active hydrocarbon-oxidizing bacteria, which can be used for bacterial preparation for the cleaning-up of the oil-polluted aquifer.
Thus, the study showed that an intensive karstification of the rock massif is one of principal natural factors that resulted in the development of oil lens at the karst water table discharging to the Kama water reservoir.
The work was financially supported by the Russian Foundation for Basic Research, project «Ural-2004», no. 040596039 and the Scientific program «Development of Science potential in Higher school» of the Federal Agency on Education at the Ministry of Education and Science of the Russian Federation, grant no. 49181.
References
- Buzmakov S.A., Kostarev S.M Human-induced changes in environmental components in oil-producing regions of Perm region-Perm: Perm univ. publishing house, 2003- 171 pp.
- Gorbunova K.A., Maximovich N.G., Kostarev V.P., Andreichuk V.N. Technogenic impact on the Karst in Perm region// Studia carstologica.-1990.-N2.-P.37–43.
- Maximovich N.G., Kazakevich S.V. Geoenvironmental peculiarities of Polazninskoe oil deposit, in: Geology and mineral resources of the Western Urals: Proceedings of the regional scientific and practical conference, Perm, 2004 — P. 277–280.
- Gorbunova K.A., et al. Karst and caves in Perm region — Perm: Perm univ. publishing house, 1992 -200 pp.
- Butyrina K.G., Gypsum karst in the central part of Perm region., Cand. Sci. (Geogr.) Dissertation, Perm, 1968.
- Gorbunova K.A., Maximovich N.G., Kostarev V.P. Technogenic activation of karst sinks in Perm region // Proceeding 7 Int. Congress Ass. of Engineering Geology, V.3. Portugal, Lisboa, 1994.-P.1929–1931.
- Pecherkin I. A., Maximovich N.G., Zakoptelov V.E. Types of cave on Kama reservoir scores // Europ. regional conf. of speleology.- Sofia, 1980. -P.34.
- Sinclair J.L., Ghiorse W.C. Distribution of aerobic bacteria, protozoa, algae and fungi in deep subsurface sediments, Geomicrobiol. J., 7, 1989 — P. 15–31.
- Kaiser J.-P., Bollag J.-M. Microbial activity in the terrestrial subsurface // Experientia, 46, 1990-P. 797–806.
- Ghiorse W.C, Wilson J.T. Microbial ecology of the terrestrial subsurface, // Adv. Appl. Microbiol., 33, 1988-P. 107–172.
- Kolbel-Boelke J., Anders E.-M, Nehrkorn A. Microbial communities in the saturated groundwater environment. II: Diversity of bacterial communities in a pleistocene sand aquifer and their in vitro activities, Microb. Ecol., 16, 1988 — P. 31–48.
- Wilson J.T., McNabb J.F., Balkwill D.L., Ghiorse W.C. Enumeration and characterization of bacteria indigenous to a shallow water-table aquifer//Ground Water, 21, 1983 — P. 134–142.
- Caldwell ME., Tanner R.S., Suflita J.M. Microbial metabolism of benzene and the oxidation of ferrous iron under anaerobic conditions // Implication for bioremediation, Anaerobe, 5, 1999- P. 595–603.
- Edwards E.A., Grbic-Galic D. Complete mineralization of benzene by aquifer microorganisms under strictly anaerobic conditions // Appl. Environ. Microbiol., 58, 1992 — P. 2663–2666.
- Elmen J., Pan W., Leung S.Y., Magyarosy A., Keasling J.D. Kinetics of toluene degradation by a nitrate-reducing bacterium isolated from a groundwater aquifer, Biotech. Bioeng., 55, 1997 — P. 82–90.
- Kleikemper J., Schroth M.H., Sigler W.V., Schmucki M., Bernasconi S.M., Zeyer J. Activity and diversity of sulfate-reducing bacteria in a petroleum hydrocarbon-contaminated aquifer, Appl. Environ. Microbial., 68, 2002-P. 1516–1523.
- Lovley D.R. Microbial Fe (III) reduction in subsurface environments, FEMS Microbiol. Rev., 20, 1997 -P. 305–313.
- Robertson W.J., Bowman J.P., Franzmann P.D., Mee B.J. Desulfosporosinus meridiei sp nov., a spore-forming sulfate-reducing bacterium isolated from gasoline-contaminated groundwater // Int. J. Syst. Evolut. Microbiol., 51, 2001 — P.133–140.
- Freeze R.A., Cherry J.A. Groundwater. Monograph, Prentice-Hall , Englewood Cliffs, New Jersey, 1979-P. 347.
- McNabb J.F., Dunlap W.J. Subsurface biological activity in relation to groundwater pollution // Ground Water, 13, 1975 — P. 33–44.
- Criddle C.S., McCarty P.L., Elliott M.C., Barker J.F. Reduction of hexachloroethane to tetrachloroethylene in groundwater// J. Contaminant Hydrol., 1, 1986 — pp. 133–142.
|
|